We believe it is possible to have lightweight buildings that extend up, above the ground, freeing ground-space for gardens, parks, agriculture, habitats, etc. Such buildings can be lightweight if they are structurally efficient. Generally, lightweight components are more expensive, so an efficient structure is one that makes optimum use of a component, justifying the extra cost.
Structural efficiency is the ability of a building to support itself and the weight of objects in it, related to the amount and type of materials required to build it. The mass of conventional large buildings is so great that most of the structural efficiency goes towards holding the building up. What if structural efficiency were increased to the point where the total mass of the building was just a small fraction of what it is now? In the last century R. Buckminster Fuller wrote extensively about this subject, and coined the term synergetic design to describe methods that are much more structurally efficient than we have today, at least for buildings. Paradoxically, we have lightweight designs for vehicles and bridges, but do not seem to be pursuing this as intensively for buildings.
The other key idea for lightweight building design is cable suspension. We already have experience with suspension bridges, and some large airports, arenas and stadiums have suspended roofs. There is a suspension building in Vancouver, B.C. called the Qube, an interesting design but still very massive. The use of high-tension cables in support allows a structure to be much more lightweight than if rigid members are used, and also helps a structure to be more earthquake resistant.
The terms "lightweight" and "cable-suspended" usually evoke ideas of flimsiness, but we believe that synergetic design can completely stabilize all degrees-of-freedom for all parts of the structure, producing a novel experience of extreme lightness combined with rigidity and stability. Actually, geodesic domes have long had this quality, covering over ground spaces, but now we get to walk around in tall structures and feel it beneath our feet.
The building design described here is just one of many possible designs. It is both synergetic and makes extensive use of cable suspension; thus it has the potential to be the lightest structure of its size ever constructed. It is also component-based; the components can be industrially mass-produced, allowing many structures to be assembled at relatively low cost. As materials technology and component technology progresses, structures constantly improve incrementally, much like the car industry has done for decades.
This "flagship" design is the source of the towerdome name, since it is composed of a rigid internal tower that cable-supports external floors that collectively have a dome (spherical) shape.
The starting point for this design is what we call the core structure. It looks somewhat like a stretched-out football, and functions as the backbone of the towerdome. All forces travel through this structure to get from the upper part down to the ground. It combines features of both the pyramid (at its top and bottom points) and the barrel (in its middle); both structures have been known since antiquity for their ability to resist compression.
The core structure functions like a cigar-shaped mast, which is wider in the middle to keep it from bending under compression. However, it is much wider, such that it not only has no tendency to bend, but it also has the geometric capability to extend out and receive and transfer forces from a wider radius. Thus, load forces from directly above tend to expand the middle outward (like a barrel being squashed) which is held inward by pentagonal rings of horizontal components (like the rings on a barrel). Forces coming in from the upper extensions tend to force the middle in the opposite direction, inwards, more or less balancing the outward forces.
When the upper extension is added to the core structure (see Images 1 and 2), we get a total of six points where weight can be supported efficiently: the center, top point of the core structure, and five points around it on the upper extension. To utilize these points we create a top level structure (see Image 3) composed of a decagon of triangles (much like a 10-piece pizza) fastened and supported at the center on the top point of the core structure, and around the edge by five triangles that extend down to the other five loadable points on the upper extension.
The top level (see Image 3) appears to be a good design, but happens to be unstable; the five support triangles have a tendency to rotate around their support points on the upper extension. To cope with this instability we add a geodesic dome (actually an "almost geodesic" dome) to go over the top level, that we call the cap dome. The cap dome appears to be just a nice covering to enclose the top level (and it is that), but it is highly structural and acts much like a bridge superstructure to transfer forces from below and provide needed stability. This is perhaps the first time a geodesic dome has ever been used in this way.
The completed tower is shown in Images 4 and 5. Of course, you may say, it does not have walls or stairs, but it is complete in the sense of being structurally complete. Such features are important, but there are many ways that they could be implemented so we are leaving that till later. At this time we are primarily concerned with the ability of the structure to be viable, and show the structure without these features getting in the way. Floors need to be shown since they are structural.
Viewing the tower structure in Image 4, one is struck with a sense of proportion and flow. It is notable that this is achieved with just 4 lengths of strut and beam components: short, medium and long beams, struts (equal in length to medium beam) and the dome strut (grey, see Image 5). After working with the icosahedral geometry for some time, we have found it to provide a large variety of interesting shapes that have an almost biological quality. We attribute this to the preponderance of the famous phi ratio, that is found extensively in nature.
Image 6 shows what we call the "force funnel"; in a completely balanced situation, all load forces are combined and conducted down to a single primary foundation point. This is very efficient, since the foundation can be a single heavy-duty earth anchor, in this case resisting being pushed down (by the weight of the building) rather than being pulled up. In an unbalanced situation, five support triangles (see Image 7) support the off-center load, and all five take some load off of the center primary support, and can support the entire structure if the center support fails.
What will keep it from tipping over? The five support triangles shown in Image 7 each have a foundation, but they also have their own earth anchor at the outer points of the base pentagon. At the left of Image 7 there is shown a set of force pathways (in red) from the earth anchor, extending up and around the core structure on three levels, forming a harness of tensile loops that completely grip the tower laterally. Later we'll see how the cable network that holds up the outer floors is also stabilized from below by these same anchors, and provides yet more lateral stability for the building as a whole.
The conclusion is yes, the suspension building is efficiently strong and very stable, and should be eminently able to withstand earthquakes. Standard buildings are very massive and thus have a lot of inertia. When the Earth moves in an earthquake, it is this inertia that puts excessive stress on the structure and tends to destroy it. The towerdome building, on the other hand, is both light and strong, so when the Earth moves, it simply moves along with it.
The tower structure has potential, we believe, to be a useful structure in its own right, in applications where a tower would normally be needed. A seventh floor can be added inside the cap dome (not shown) that is big enough to accomodate a vertical axis wind turbine. It could be very quiet and generate good power, since wind speeds would be greater 56 feet above the ground. When wind speed is doubled it generates eight times the power, a big advantage. Water can also be stored in the pentagonal pyramid on level 5 (at the top of the core structure), and the large mass of the water can help to dampen vibrations in the structure.
Next, we explore the possibility of creating suspended outer floors. Due to the icosahedral geometry of the towerdome system, it is possible to surround the tower on levels 2 to 5 with "golden triangles" that are rigidly attached to the tower on the inside (see Image 8), but on the outside are suspended by a cable network. This greatly increases the amount of floor space in the building.
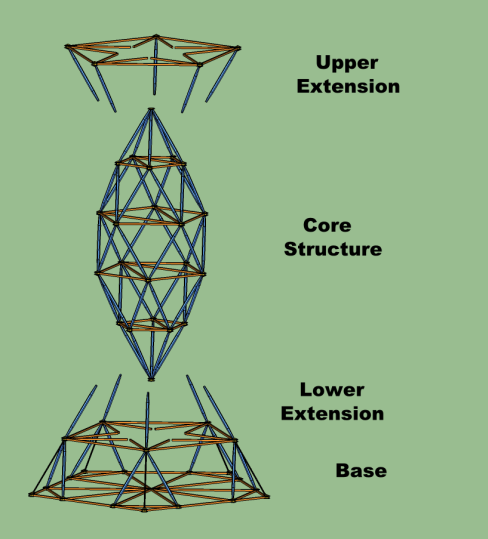
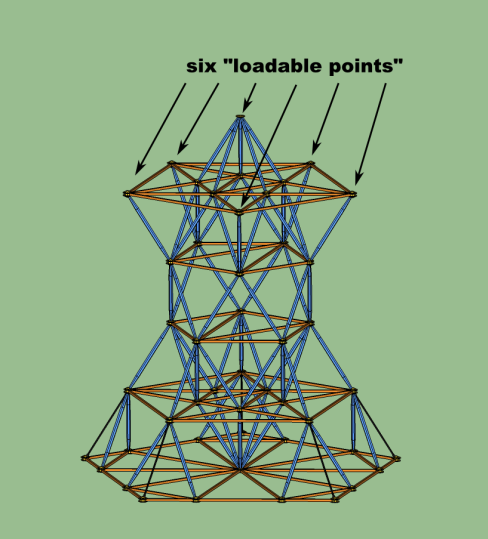
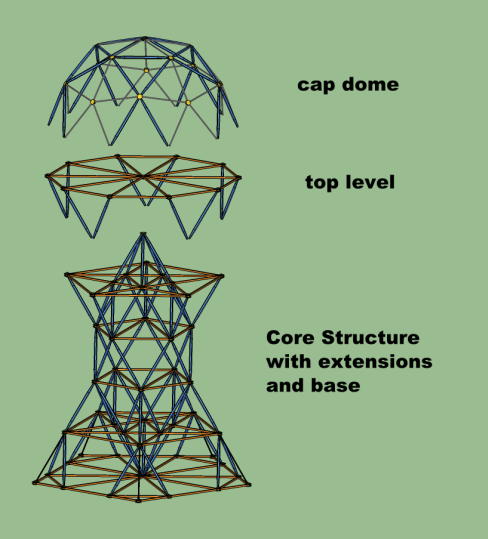
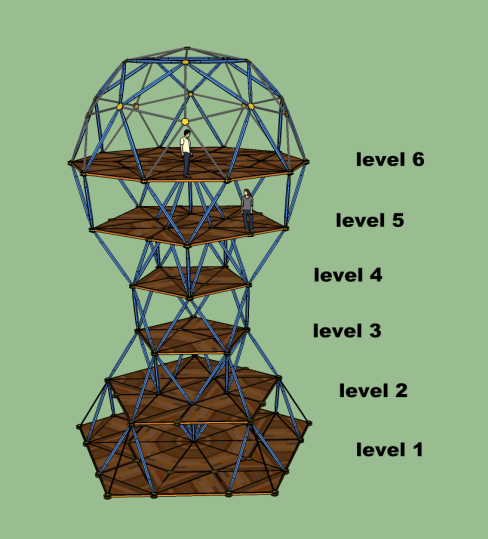

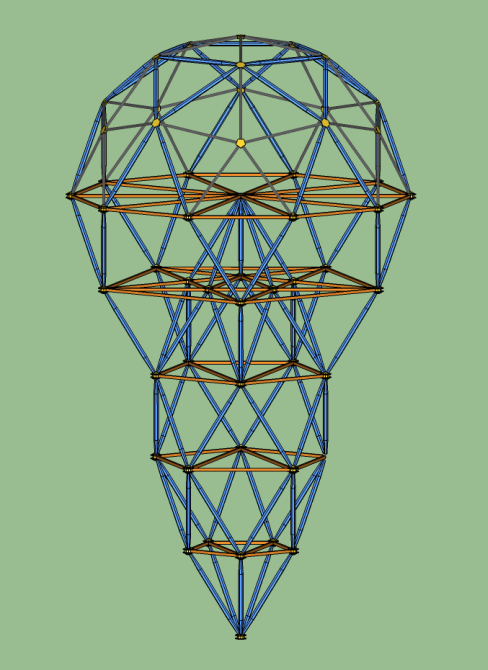
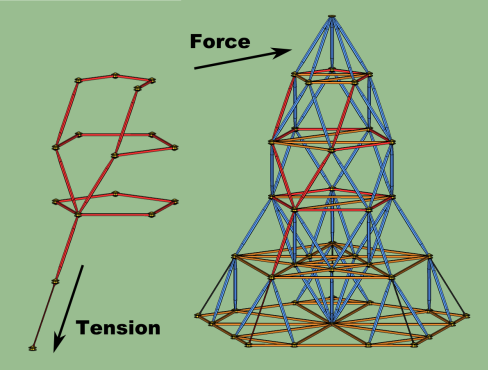
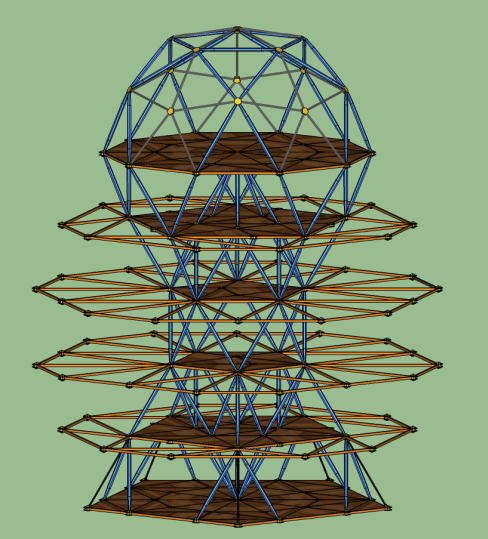
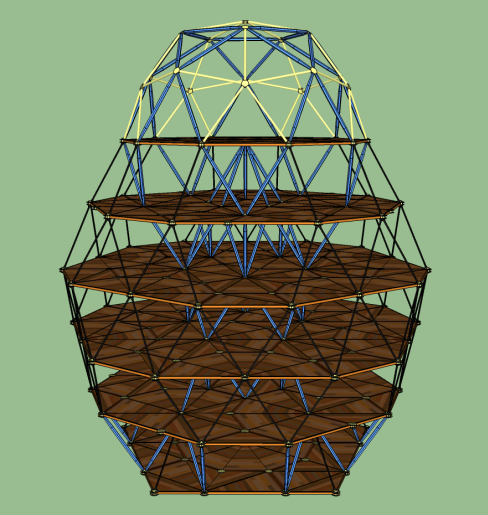
These are the smallest levels in the building, but they are also the most open. On the ground floor (level 1), the building could be left completely open, perhaps in an eco-sensitive area for minimum impact to the environment. The top floor (level 6) is very similar to a standard geodesic dome, except that it is elevated to more than 40 ft above the ground, for excellent panoramic views.
These levels are tiled by 20 LSL areas and 10 SLS areas, 772 ft² (71.7m²) each.
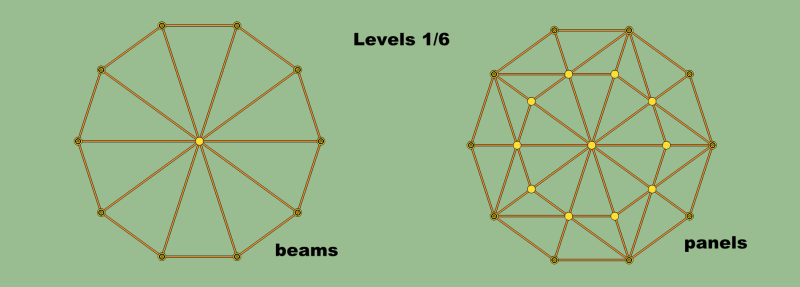
These levels are much larger than levels 1 and 6, but not as large as levels 3 and 4. They are also the least open, with a lower percentage of usable floor space. Because of this they might be appropriate for utilities and storage, although they should be able to provide five good-sized rooms apiece.
These levels are tiled by 31 LSL areas and 22 SLS areas, 1315 ft² (122m²) each.
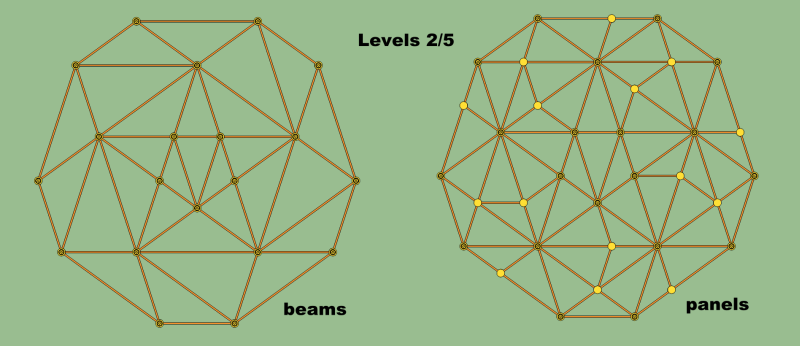
These are the largest levels in the building. Even one level, say level 4, could be large enough for a family living space, with bedrooms upstairs on level 5. Level 3 could then be used for office and activities space.
These levels are tiled by 44 LSL areas and 23 SLS areas, 1716 ft² (159m²) each.
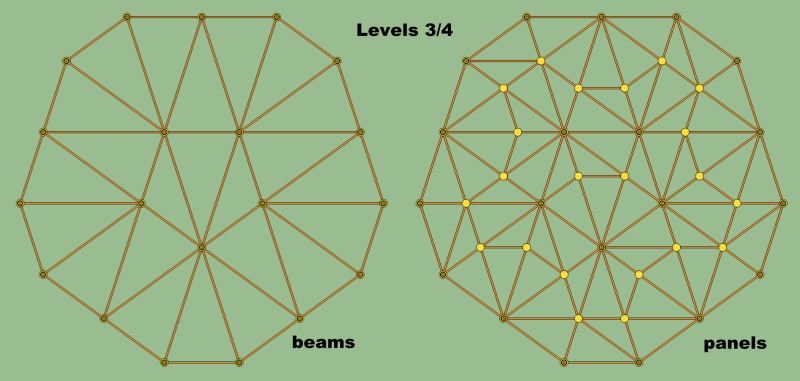
Lightweight buildings - conclusion
This is the initial Towerdome suspension building design. Of course, many other designs are possible. The building is supported by an internal rigid tower; the outer floors are suspended from the top of the tower by a network of cables that helix around in both directions and are fastened on the bottom at five earth anchor support points. The building has 7600 ft² (706 m²) of floor space on six levels; the ground level is 772 ft² (71.7 m²), an amazing 11% footprint. The top level is covered by a structural geodesic "cap dome" that stabilizes it.
